Originally published on December 4, 2020 by BNP Media through the Building Enclosure Blog.
The building sector accounts for about 40% of global carbon emissions
Over the summer, the
AIA published its Climate Action Plan and urged the building design and construction industry to act quickly to mitigate the building sector’s atmospheric carbon contribution.
Solutions toward this end may take a variety of forms – not the least of which being new zero-carbon, high-performance buildings that provide ongoing operational energy savings and risk mitigation.
However, in the U.S., the vast majority of the building stock we will have 10 years from now already exists – and many of those buildings are energy sieves.
Data from the Energy Information Administration (EIA) indicates that nearly half of the U.S. commercial building stock was built before 1980; moreover, over 70% of the U.S. commercial stock was constructed before 1990.
Existing buildings and embodied carbon
Every phase of a product’s lifecycle comes with an energy and carbon consequence. The carbon load released to the atmosphere through the manufacturing, production, transportation, installation, and disposal of our building materials is referred to as
embodied carbon. When we reuse an existing building, not only is the embodied carbon of demolition spared but so is the embodied carbon of the new foundation, structure, and envelope. Using today’s standard practices, renovating an existing building can result in embodied carbon being
as low as 10-20 lbs/sf – roughly a sixth of that of a new large, heavy (e.g., concrete and/or steel structure) building.
Existing buildings and operational carbon
Operational carbon is the carbon load created by the use of energy to heat and power a building. It is dependent on a building’s energy consumption, which is something that is constantly happening and always in flux – like a water faucet with the valve constantly being adjusted. Calculating operational carbon is complicated because depending on the location of a building, its mechanical and electrical systems, and a variety of other factors, the ultimate source(s) and quantity of energy consumption and related carbon emissions may vary significantly.
However, the ultimate solutions (and their priority) are simple:
- Reduce energy consumption.
- Use on-site renewable energy production.
- Purchase green power from the utility.
Do not expect a “silver bullet” solution for retrofits
When it comes to existing buildings, the highest priority – reducing energy consumption – can be the most complicated. Within the constraints of an existing building, every energy conservation measure (ECM) will come with a specific cost-effectiveness, energy impact, and operational carbon consequence. Project teams would be wise to invest in retro-commissioning services, which will entail a rigorous analysis of the building’s performance and the impacts from a variety of potential ECMs. Expect an evidence-based discovery process. What “pencils out” on one project may not make sense in another existing building condition.
One of the best high-profile examples of a deep energy retrofit is the Empire State Building in New York City. Built in 1930, it was not originally designed in accordance with today’s high-performance building solutions. Yet, over the past decade the high-rise has undergone a ground-breaking deep energy retrofit as part of the
$550 million Empire State ReBuilding restoration program.
Through a major envelope and mechanical upgrade effort led by the Empire State Building Company, LLC, the Clinton Climate Initiative, Jones Lang LaSalle, Johnson Controls, Inc., and the Rocky Mountain Institute, over 60 energy efficiency measures were assessed as part of a comprehensive analysis. An iterative energy, financial, and carbon emissions modeling process identified 8 implementable ECMs which combined to reduce the annual energy use of the Empire State Building by approximately 38% (see figure).
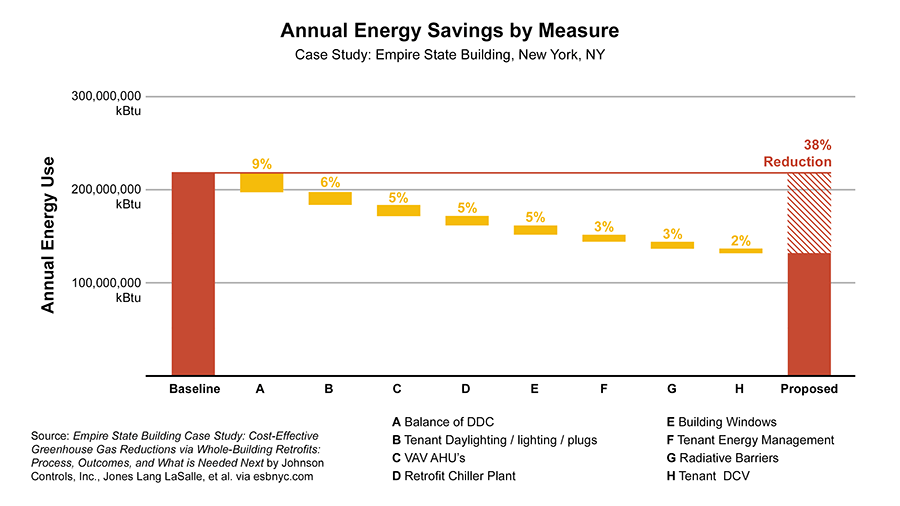
Figure: Annual Energy Savings by Measure for the Empire State Building.
Adapted from: Empire State Building Case Study: Cost-Effective Greenhouse Gas Reductions via Whole-Building Retrofits: Process, Outcomes, and What is Needed Next by Johnson Controls, Inc., Jones Lang LaSalle, et al. via esbnyc.com (accessed December 1, 2020 at https://www.esbnyc.com/sites/default/files/ESBOverviewDeck.pdf).
Aside from the balancing of the direct digital control (DDC) system, which entailed upgrading the existing controls systems throughout the building and offered a 9% impact, the average energy use reduction of the other ECMs in the bundle was just over 4%. This information underscores that the impact and cost-effectiveness of energy conservation measures will be unique to any existing building and warrants rigorous analysis. Moreover, it will likely require a bundle of energy conservation measures to realize deep energy and carbon impacts but significant reductions are possible.
Investing in our existing building stock is critical. Without doing so, we will never effectively reduce the carbon emissions from the building sector.
More on energy efficiency and buildings
Toward a Decarbonized Built Environment
How to Make Building Energy Models More Accurate
Stages of Design and Types of Energy Models